- HOME
- 刊行物のご案内
- 心理学ワールド
- 104号 空間認知の科学 最前線
- ヒト以外の生物における ナビゲーション─振動子と働くサーボ機構
【特集】
ヒト以外の生物における ナビゲーション─振動子と働くサーボ機構
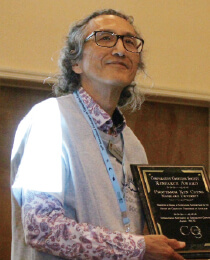
ケン・チェン(Ken Cheng)
Profile─ケン・チェン
カナダのトロント大学卒業。米国ペンシルバニア大学で博士号取得後,トロント大学等での研究員を経て,1995年,オーストラリアのマッコーリー大学に着任,現在に至る。これまでナビゲーションの認知的メカニズムに関する研究を,ヒト含む脊椎動物から無脊椎動物まで幅広く行ってきた。Journal of Experimental Psychology: Animal Learning and Cognition誌などに論文多数。著書に“How animals think and feel: An introduction to non-human psychology”(単著,Greenwood)など。
『動作の体制化』(“The Organization of Action”)[1]の中で,ガリステルは,動作(action)の3つの基礎構成要素である反射(reflex),振動子(oscillator),サーボ機構(servomechanism)について述べている。反射は,「適切な刺激」と呼ばれる特定の刺激群によって確実に引き起こされるステレオタイプ的な動作,振動子は,振動と呼ばれる規則的・周期的な動作を生み出す内因的メカニズム,そしてサーボ機構は,連続的に働き続ける誤差修正装置を指す。コンパレータ(comparator:比較器)や積分センター(integration centre)と呼ばれる内的装置は,センサー入力から得られた変数の,現在の読み取り値をその変数の「望ましい」設定値と比較する。観察された現在の値と設定値・参照値の差分が「誤差」(error)で,誤差を低減させるフィードバックループ(negative-feedback loop)の中で,サーボ機構が動作を引き起こす。反射とは異なり,サーボ機構における動作は,最初にそのメカニズムを動かした入力値を必ず変化させることになる。最近,私は,振動子とともに作動するこのサーボ機構が,マイクロメートルレベルから数千キロまでのあらゆる範囲の移動について,生物によるナビゲーションの普遍的な枠組みを形成している,という提案をした[2, 3]。
ナビゲーション(navigation)とオリエンテーション(orientation)は,いずれもゴールに向かって移動することだが,私はこの2つを区別している。オリエンテーションは,ある「よい」場所に向かって移動することで,つまり,特定の目的地ではなく,「よりよい」か「より悪い」かが基準になっている。細菌や寄生虫が,食べ物を示して徐々に濃くなる化学物質を遡っていくのが,その例だ。一方,ナビゲーションは,ある特定の場所に向かって移動することで,例えば,ミツバチやアリが,(他の巣ではなく)自身の巣に向かって移動することが挙げられる。この区別にかかわらず,オリエンテーションとナビゲーションの双方において,振動子と協働するサーボ機構が移動する生物を動かしている。
ナビゲーションに関わるサーボ機構が,移動する生物を目的地に向かわせる。すなわち,移動方向が目的地方向からずれると「誤差」が生じ,負のフィードバックループにおいてこの誤差を低減するように動作が引き起こされる[4, 5](図1)。目的地方向の設定は,ナビゲーションのメカニズムによって異なる。経路統合(経路積分:path integration)においては,現在地から出発点(典型的には居住地/巣)までの距離と方向を符号化したベクトルの形で与えられ[6],風景に基づくナビゲーションでは,移動における目指すべき特定の風景の形で与えられる。私は,この考えを四半世紀以上維持してきた[4]。しかし最近,洗練されているものいないもの,形は違っても,振動子と協働するサーボ機構が移動をガイドする上で働いていることを発見した[2, 3](図1)。ここで例を出して説明するのがよいだろう。スキーマの導入を論じる前に,極小さいものから大きなものまで,いくつかの事例に焦点を当てる。
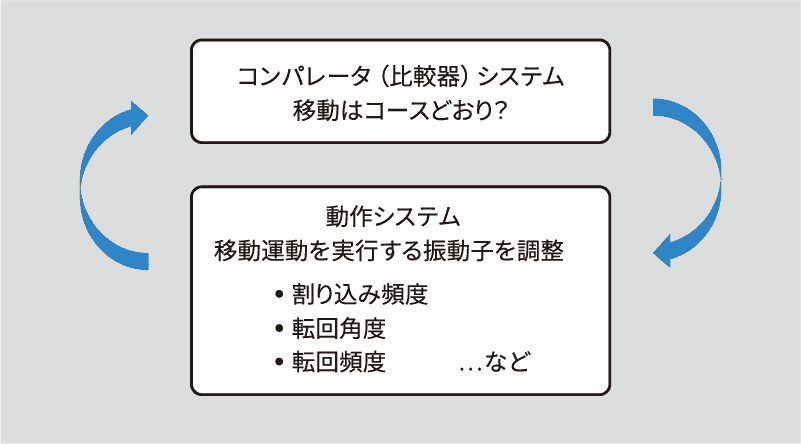
コンパレータ(比較器)は,コースを保つために,現在の感覚・知覚情報を目標情報と比較し,移動運動を実現する振動子(鞭毛や繊毛,翼,四肢)を調整する。
事例紹介
オリエンテーションの単純で最も広く見られるかたちは,移動運動(locomotion)を実行している振動子の信号に時々割り込んで移動方向を変えることだ。大腸菌(Escherichia coli)は単純な細菌と考えられているが,それでも30種ほどの化学受容器を備え,化学物質のグラデーションを遡ったり離れたりする走化性(chemotaxis)で知られている[2, 7, 8]。この微生物は,5本程度の鞭毛を振動させながら移動する振動システムを持っている。モーターがその鞭毛を一方向(例えば,反時計回り)に動かすと,すべての鞭毛が束になって1本の長い尾のようになり,精子の尾のように振動することで前進する。時折モーターが逆方向(ここでは,時計回り)に鞭毛を動かすと,すべての鞭毛がバラバラになって振動機構に割り込み,前進を止める。大腸菌は,ランダム方向にスピンするが,モーターが安定した転回を再開すると,新たにランダムに選ばれた方向に大腸菌は移動していく。
このようなランダムな方向転換が,どうしてゴールに向かう運動につながるのか? それは,このような振動機構への「割り込み」率を調整することによって可能になっており,ケモキネシス(chemokinesis)と呼ばれている。キネシスは,ある入力に基づいてある行動の割合を調整するメカニズムを指すが,平均して「よりよい」方向に進ませるものではない。文献的には,このような過程のケモキネシスは,走化性と呼ばれることが多い。ルールは単純で,「よりよい」方向に向かっているときは,方向転換の割合が減少する。逆によりよい方向に向かっていないときは,方向転換の割合が増える[2, 7]。大腸菌は,化学物質濃度の現在値に適応しているため,移動にともなうわずかな濃度変化を検出できる。大腸菌は,小さすぎてその体のどちら側の化学物質濃度が濃いかを検出できないため,最長1秒のわずかな時間前の濃度の記憶を使う。このメカニズムによって大腸菌は,化学物質濃度がそれ以上よくならないピークまで遡っていき,その場所でくるくる動き回るのをやめる。この単純なスキーマは十分機能するため,ある研究者は,コンピュータゲーム上で大学生にこの戦略を採用させることができた[9]。その大学生たちは,大腸菌と同じように,目的とするグラデーションのピークまで遡ることに成功した。
ランダムな方向転換の割合を調整するメカニズムは,小さな生き物には共通している。移動運動を可能にする効果器は異なっていても,本質的には同じサーボ機構が,細菌とは別の原核生物群である古細菌(Archaea)の走化性においても使われている[10]。アーキア鞭毛(archaella)と呼ばれる古細菌の効果器は,大腸菌のような細菌を動かす鞭毛とは進化的に起源が異なっているが,古細菌のケモキネシス機構の一部は,細菌から拝借したと考えられている。ケモキネシスは,ゾウリムシ(Paramecium)といったはるかに大きな(しかし,やはり単一細胞の)真核生物[11]や線虫(Caenorhabditis elegans)[12]でも採用されている。大腸菌が手こぎボートだとすれば,ゾウリムシは,大きなクルーズ船ほどの大きさだ。ゾウリムシは,時折バックし,ランダムな方向にスピンする。これは,ゾウリムシが何かにぶつかったときに見せる行動なので,回避反応と言われている[8, 11]。線虫は, 302のニューロンからなる小さな神経システムを備えているが,移動運動のための神経と筋肉に加え,側方(つまり左右の)振動に基づくより強力なメカニズムを見出すことができる。これこそがオリエンテーション機構における大きな進化的転換点かもしれない。
複数の振動子が互いに協調して筋肉を駆動し,線虫を左右にくねらせる[8]。筋肉は背側と腹側にあるが,それぞれの収縮が左右方向のくねりに変換され,結果線虫は側方に転回する。化学物質の空間的なグラデーションを検出するには体サイズの小さすぎるゾウリムシや大腸菌[8]とは異なり,左右への振動によって十分に空間的に分離された状態で,線虫は体の左右の化学物質濃度を比較できる[13]。この左右比較により,線虫はさらに左もしくは右に転回できる。側方振動により,生物は,真の走化性,つまりランダムに方向を変えるのではなく,平均して,進むべき「よりよい」方向に向くこと,を可能にする。これに対して,ケモキネシスは,すでに述べたように,ある行動の確率を変化させるに過ぎない。ショウジョウバエ(Drosophila)の幼虫は,この真のタイプの走化性を持っている[14]。左右への振動は,空間的に化学物質濃度の左右比較を可能にし,それに従って,転回角度を調整する。
ナビゲーションしているアリの最近の観察では,このよく研究されてきたナビゲーション生物がやはり,6本脚の通常の振動に加えて,左右に振動することが明らかになった[2, 15, 16]。この左右への振動は,ナビゲーションのために調整される。例えば,私たちが「確信のなさ」(uncertainty)と呼んでいるものを増大させるような操作によって,より頻繁でより大きな振動が引き起こされる。すなわち,この状況下では,アリは,「蛇行」(meander)しながら移動する。例えば,既知のルートを通って巣に戻ってきたアリをルート途中のどこかに戻すと,次に巣に戻るとき,アリはより蛇行するようになる[17]。私の研究グループによる未公刊の最近の観察では,巣までの通常の風景を変えてやることでも,より多くの蛇行につながることが示唆された。「確信のなさ」は,定義があいまいで,擬人的な言い方かもしれない。アリがこのような「確信のなさ」に対応するものとして何を符号化しているか明らかではない。「確信あり」と「確信のなさ」は,視覚風景やその他感覚手がかりがそれぞれ既知か未知かを反映しているに過ぎないかもしれない[16, 18]。
アリは,パノラマ的視覚風景を手がかりとしてナビゲーションに用いる[19, 20]。視覚風景は移動中の生物にゴール方向に対して極端に左または右を向いていないかという情報を与えるため,左右への振動は移動方向を常に最適方向に保つよう調整し続けてくれる,というのが左右振動が生み出されることの合理的な説明だ[16, 21]。このトピックについては,さらなる実験や理論的研究が必要である。
地球規模では,ウミガメが,地磁気手がかりを使いつつ広い安全地帯に留まりながら大洋を旅する[2, 20, 22]。ウミガメは,磁場の伏角(地球地表の水平方向に対する磁場の角度)と磁気の強さを符号化することで,世界の「どこにいるか」という感覚を得ている。括弧つきなのは,彼らは自分たちを特定の緯度・経度に位置づけていない可能性があるからだ。実際,ウミガメのナビゲーションの研究の多くを生み出しているグループ[20, 22, 23]は,ウミガメたちがある特定の地磁気条件のときに,単にどちらに向かうべきかを知っているだけで,安全地帯を回る多数のルートを知っているだけ,という方がはるかに近い,と考えている。しかし,問題は,彼らが向かいたい方向に進み続けるのに波が障害となることである。
波によって,ウミガメは,ヨー(yaw:左右転回方向),ピッチ(pitch:頭の上げ下げ方向),ロール(roll:前後軸に沿う回転方向)の3回転平面で,常に進みたい方向から逸らされてしまう。ウミガメは,バタフライのように,シンクロして振動する前ひれのパワー・ストロークによって泳ぐ[24]。振動するひれと協働するサーボ機構は,ロール・ピッチ・ヨー方向の回転に対して進行方向を調整する。例えば,ロール方向の回転に対し,前ひれの左右で異なる深さで水をかくことで,体のバランスを取る。ウミガメを彼らの自然生息域である大洋で追跡するのは困難であるため,私がサーボ機構と呼んでいるこれらの研究は実験室で実施されるが,将来,自然条件下で波に直面したときに彼らがどのように調整しているか調べる挑戦には意味があるだろう。
考察
このように,すべてのスケールを通して,移動する生物においてサーボ機構が振動子とともに働いている[2, 3, 20]。これらのメカニズムは,洗練さにおいて多様だ。ケモキネシスにおける前進運動への割り込みは,移動運動を駆動する振動子を時々止める以上のことはしていない。大きな進化上の「発明」は,側方振動の形で現れた。すなわち,側方振動により,生物は,ランダム方向ではなく,左か右に向き直って「よりよい」方向に向かって進めるようになったのである。このような側方振動では,生物個体のサイズが大きくなり,神経や筋肉を必要とするかもしれないが,これはさらに調べていく価値のあるトピックであろう。最も洗練されたレベルでは,より不確実と考えられる条件においてより振動するなど,サバクアリは環境の状況に応じてさまざまな方法で振動を調整する。
私の考えでは,振動子は,オリエンテーションやナビゲーション以外でも働いている。振動子は,生活においてあまりにも当たり前なので,サーボ機構とともに生活の基本構成要素とも言えるだろう。他の認知の領域でも,課題を達成するために振動子を調整している。哺乳類の脳は,θやγといったさまざまなギリシア文字で知られる,認知に関わる固有のリズムがあることが特徴である[25]。注意や,知覚,記憶といった多くの種類の認知行動が周期的に「満ち欠け」する[3]。複雑な脳は,振動周期なしに,その諸活動を体制化する(日常的な言葉で言えば,ともに動作する)ことはできないのだろう[26]。複数の核を持つが,単一細胞の「知性的な」粘菌(Physarum polycephalum)も振動に基づいて生活している。粘菌中の細胞質は,行ったり来たりかき回されており,そのような振動を調整することで,食物を探したり,好ましくない化学物質を避けたり,意思決定したり,その他知的な課題をやってのけているようだ[27]。
サーボ機構もまた,生活でありふれており,サーボ機構なしに生きていくことは不可能だ,とまで言わせてもらおう。心理学のさまざまな研究者が,ヒトの認知と動作が,サーボ機構的な方法で働いている,という見方を打ち出している[28, 29, 30]。生理学では,生理学の中心テーマとも言うべきホメオスタシスを維持する上でのサーボ機構の役割を強調している[31]。「なぜ,なんのために」という問いに対して私なりに短くまとめるなら,内的生理機構を含む自身の活動を体制化するために振動子は必要であり,世界の環境に適応するためにサーボ機構は必要である,ということだ[2, 3]。サーボ機構と振動子は動作の基礎構成要素やナビゲーション能力の構成要素であるだけでない。生きていく上での基本構成要素なのである。
(訳:牛谷智一)
訳者追記
本稿は,本誌の性質を考慮し,英語で書かれた原文の意を損なわない限りにおいて,自然な日本語となるよう訳した。そのため,原文にはない括弧等を用いている。なお,英語原文は,本記事のウェブサイトにて読むことができる。
本稿を通じて使用されている「振動」は,物体がガタガタ音を立てているときなどに使用されるような,日常的かつ狭い意味ではなく,冒頭に書かれているように,左右や上下といった方向に,規則的に周期的に「往復」する運動という,より広い意味で使われている。ばねに重りを付けて引っ張って離した際の,重りの動きを想像していただきたい。高校物理に出てくる「ばねの単振動」の「振動」である。
Navigation in non-human organisms: Servomechanisms working with oscillators
School of Natural Sciences Macquarie University
Sydney NSW 2109, Australia
In a book called The Organization of Action, Gallistel (1980) described three basic units of action: the reflex, oscillator, and servomechanism. The reflex is a stereotypical action triggered reliably by a particular class of stimuli called adequate stimuli. An oscillator is an endogenous mechanism for generating regular periodic action, oscillations. Oscillations are the periodic actions and the oscillator is the system that generates them. A servomechanism is an error-correcting device that operates continuously. An internal device known as a comparator or integration centre compares a ‘desired’ setting on a variable to the current reading of that variable as supplied by the senses. The difference between the observed current value and the setting or reference value on the variable constitutes error. Error drives action in a servomechanism in a negative-feedback loop that reduces the error. In a servomechanism, unlike a reflex, the action must change the very input that drives the mechanism in the first place. I have recently proposed that servomechanisms working with oscillators constitute a universal paradigm across life for navigation by mobile organisms, from micrometres to thousands of kilometres of travel (Cheng, 2022, 2023).
Navigation and orientation move a traveller towards a goal. I distinguish the two terms. In orientation, the traveller is aiming to get to a better place, by some criteria of better and worse, but not to any particular goal. A bacterium or worm ascending a chemical gradient signaling food is an example. In navigation, on the other hand, the traveller is aiming to get to one particular place. A honeybee or ant trying to get back to its own nest, and not just any nest, is an example. In both orientation and navigation, servomechanisms working with oscillators propel the traveller.
A navigational servomechanism works to aim the traveller towards a goal. Any deviation from the goal direction constitutes an error, and the error drives action to reduce the error in a negative feedback loop (Cheng 1995, 2012; Figure 1). Different mechanisms code the goal direction in different ways. In path integration, the goal direction is specified by a vector encoding the direction and distance to the starting point, typically the home (Wehner 2020). In view-based navigation, the goal direction is specified by a particular view to aim at in travelling. I have maintained this conception for more than a quarter of a century now (Cheng, 1995), but recently, I have found that navigational servomechanisms work with oscillators, in more or less sophisticated ways, in guiding travel (Cheng 2022, 2023; Figure 1). At this point, examples best illustrate. I will highlight a few cases from the very small to the very large, before discussing the import of this scheme.
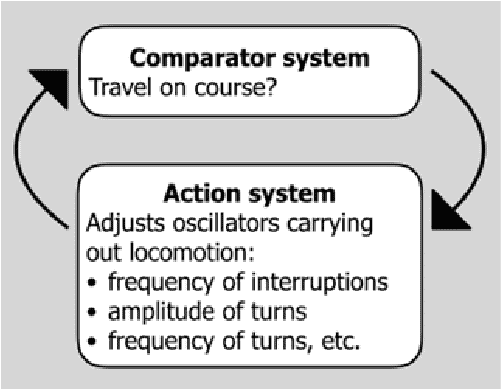
A comparator system compares current sensory and perceptual information with goal information and adjusts oscillators carrying out locomotion to keep on course, oscillators such as flagella, cilia, wings, and limbs.
Illustrative cases
Perhaps the simplest and most widesspread mode of orientation is to occasionally interrupt the oscillators carrying out locomotion, and change direction of travel. The bacterium Escherichia coli is considered a simple bacterium, but it still possesses some 30 kinds of chemoreceptors and can go up or down a chemical gradient, a process known as chemotaxis (Koshland 1980; Sterling and Laughlin 2015; Cheng 2022). The microbe moves by beating its ~5 flagella in an oscillatory system. When the motors driving the flagella turn in one direction, all the flagella bundle up into one long tail that oscillates like the tail of a sperm, and the bacterium moves forward. Occasionally, the motors turn the other way, and all the flagella come apart, interrupting the oscillations and the forward movement. The organism takes a spin that orients it in a random direction. When the motors resume steady turning, the microbe travels in this new, randomly chosen direction.
How can occasional random changes of direction achieve goal-directed movement? By adjusting the rate of such interruptions, a process called chemokinesis. A kinesis is a mechanism that adjusts the rate of some behaviour based on input; it does not on average pick a better direction of travel. In the literature, this process of chemokinesis is often called chemotaxis. We can say that chemokinesis is one variety of chemotaxis. The rule is simple: When the going is getting better, the rate of direction change is reduced; when the going is not getting better, the rate of direction change increases (Koshland, 1980; Cheng, 2022). The bacterium adapts to the current level of chemical concentration so that it can detect small changes in concentration as it moves. It is far too small to detect the difference in chemical concentration across its tiny body. It thus has use a memory of the concentration a short time (up to 1 second) ago. With this mechanism, the bacterium ascends to a peak of chemical concentration, where things do not get any better, and the organism ends up milling around there. This scheme works well enough that two scientists could force undergraduate students to adopt this strategy in a computer game (Marken & Powers, 1989), and the students, like E. coli, succeeded in ascending to the peak of a goal gradient.
The mechanism of adjusting the rate of random direction changes is common in small organisms. While the effectors that drive locomotion are different, essentially the same servomechanism is used in chemotaxis by the domain of Archaea, another branch of prokaryotes besides bacteria (Quax, Albers, & Pfeiffer, 2018). The effectors that drive Archaea, called archaella, have a different evolutionary origin from the flagella that propel bacteria like E. coli, but Archaea are thought to have borrowed some of the machinery for their chemokinesis from bacteria. Chemokinesis is also practiced by much bigger but still single-celled eukaryotes such as Paramecium (van Houten 1978), and by the nematode Caenorhabditis elegans (Srivastava et al. 1999). If E. coli were a small sailboat, Paramecium would be a big cruise ship. Paramecium occasionally backs up and spins in a random direction, a process called an avoidance response because that is what the eukaryote does on bumping into something (van Houten, 1978; Sterling & Laughlin, 2015). The worm C. elegans sports a small nervous system of 302 neurons, and with nerves and muscles for locomotion, we find an additional, more powerful mechanism based on lateral (left-to-right) oscillations, perhaps a major evolutionary transition in orientational mechanisms.
Oscillators coordinating with one another drive the muscles of C. elegans to make the worm ‘wiggle’ right and left (Sterling & Laughlin, 2015). The muscles are located dorsally and ventrally, but the worm turns on its side, so that dorsal and ventral contractions turn into left-right wiggles. With enough spatial separation now arising from the lateral oscillations, the nematode can compare the chemical concentration on the right and left sides (Iino & Yoshida, 2009)—unlike Paramecium and E. coli, for which the spatial chemical gradient is far too small to detect (Sterling & Laughlin 2015). On the basis of the left-right comparison, the worm can turn more to the left or right. Lateral oscillations allow an organism to execute true chemotaxis, in which the directional change is not random but, on average, leads to a better direction for travel. As noted, chemokinesis, in contrast, only changes the rate of some behaviour. Drosophila larvae also use this form of true chemotaxis (Wystrach, Lagogiannis, & Webb, 2016). Lateral oscillations allow them to compare spatially the chemical concentrations on the left and right sides, and adjust their turn sizes accordingly.
Recent observations of navigating ants show that these much-studied navigators also oscillate left and right, on top of their regular oscillations of their 6 legs (Murray et al. 2020; Cheng 2022; Clement et al. 2023). These oscillations are adjusted in the service of navigation. For example, any manipulation that increases what we would colloquially call uncertainty leads to more and bigger oscillations: ants ‘meander’ more in such circumstances. If an ant is picked up after it has travelled a familiar route home and placed back somewhere on its route again, that increases the meandering on the next run home (Wystrach, Schwarz, Graham, & Cheng, 2019). Recent, as yet unpublished observations from my group suggest that a change in the usual view home also leads to more meandering. “Uncertainty” is an informal and perhaps anthropomorphic term. It is still unclear what ants encode by way of such uncertainty. Perhaps, it reflects familiarity (certainty) vs. unfamiliarity (uncertainty) in their views and other sensory cues (Baddeley et al. 2012; Clement et al. 2023).
Ants use panoramic views in navigation (Wystrach et al. 2011; Freas & Cheng, 2022). One plausible mechanism generating lateral oscillations is that views inform the navigator whether they are too far to the left or right of the goal heading, so that the lateral oscillations keep adjusting the direction of travel to keep to the best direction (Clement et al., 2023; Wystrach, 2023 preprint). More empirical and modelling work are needed on this topic.
On a global scale, sea turles roam the oceans of the world, staying within a large safe zone using geomagnetic cues (Lohmann & Lohmann, 2019; Cheng, 2022; Freas & Cheng, 2022). They code the inclination (the slope of the geomagnetic field with respect to the horzontal surface of the Earth) and the intensity of the magnetic field to obtain a sense of ‘where they are’ in the world. The phrase in quotes points to the fact that the turtles may not be locating themselves at a particular longitude and latitude in the world. In fact, the group that produced most of the research (Putman et al. 2011; Lohmann & Lohmann, 2019; summary: Cheng & Freas, 2022) posit that the turtles simply know which directions to head in when given particular geomagnetic conditions, far more like knowing a bunch of routes around their safe zone. Waves, however, give them problems in keeping to their desired heading.
Waves constantly knock them off their desired course of travel in all three rotational planes, yaw (left-right), pitch (head up and down), and roll (around the front-to-back axis). Sea turtles swim by power strokes of the front flippers, oscillating in syncrhony like the butterfly stroke (Avens et al. 2003). Servomechanisms working with oscillating flippers adjust the turtle’s course of travel against rotations in roll, pitch, or yaw. Against rolls, for example, the two front flippers stroke at different depths to rebalance the body. These studies of what I am calling servomechanisms are conducted in the lab, it being difficult to track sea turles in their natural ocean habitat. But it is well worth the effort to investigate their adjustments in the face of waves in natural conditions.
Discussion
Thus, across all scales of travel, servomechanisms work with oscillators in navigating organisms (Cheng, 2022, 2023; Freas & Cheng, 2022). These mechanisms differ in sophistication. Interruptions of forward movement in chemokinesis do nothing more than occasionally stopping the oscillators that are driving locomotion. A big evolutionary ‘invention’ came in the form of lateral oscillations, which allow an organism to adjust turns to the left and right and pick a better rather than a random direction of travel. Such lateral oscillations require a scale up in size and perhaps neurons and muscles, a topic well worth investigating further. At the sophisticaled end, desert ants adjust oscillations in various ways in response to circumstances, such as oscillating more in what can be considered more uncertain conditions.
My view is that oscillators serve much more than orientation and navigation. They are so common in life that they, along with servomechanisms, are likely basic units of life. Other realms of cognition too adjust oscillators to accomplish tasks. Mammalian brains are characterised by intrinsic rhythms that serve cognition, known by various Greek letters such as theta and gamma (Buzsaki, 2019). Many kinds of cognitive performance, in attention, perception, and memory, waxes and wanes in cycles (Cheng, 2023). A complex brain may not be able to organise its activities, informally, get its act together, without oscillating cycles (VanRullen, 2016). The multi-nucleated but single-celled ‘intelligent’ slime mould Physarum polycephalum lives on oscillations. Its entire cytoplasm sloshes back and forth, and adjustments to such oscillations allow it to search for food, avoid nasty chemicals, make decisions, and do other seemingly smart tasks (Reid, 2023).
Servomechanisms are also common in life, and I venture to say that life is not possible without servomechanisms. Various scholars in psychology have put forth the view that human cognition and action operate in servomechanistic fashion (Miller et al., 1960; Powers, 1973; Carver & Scheier, 1998). Physiology features servomechanisms in maintaining homeostasis, perhaps the central theme in physiology (Stanfield, 2016). My brief summary on the why and wherefore is that oscillators are needed to organise one’s own activities, including internal physiology, while servomechanisms are needed to adjust to circumstances in the world (Cheng, 2022, 2023). Servomechanisms and oscillators are not just basic units of action and basic components of navigation. They are basic units of life.
- 1.Gallistel, C. R. (1980) The organization of action: A new synthesis. Erlbaum.
- 2.Cheng, K. (2022) Proc R Soc Lond B Biol Sci, 289, 20220237.
- 3.Cheng, K. (2023) Anim Cogn, 26, 73-85.
- 4.Cheng, K. (1995) Landmark-based spatial memory in the pigeon. In D. L. Medin, Ed., The psychology of learning and motivation (Vol.33, pp.1-21). Academic Press.
- 5.Cheng, K. (2012) Arthropod navigation: Ants, bees, crabs, spiders finding their way. In T. R. Zentall & E. A. Wasserman, Eds., The Oxford handbook of comparative cognition (pp.347-365). Oxford University Press.
- 6.Wehner, R. (2020) Desert navigator: The journey of an ant. Belknap Press.
- 7. Koshland Jr, D. E. (1980) Annu Rev Neurosci, 3, 43-75.
- 8.Sterling, P., & Laughlin, S. (2015) Principles of neural design. MIT Press.
- 9.Marken, R. S., & Powers, W. T. (1989) Behav Neurosci, 103, 1348-1355.
- 10.Quax, T. E. F. et al. (2018) Emerg Top Life Sci, 2, 535-546.
- 11.Van Houten, J. (1978) J Comp Physiol, 127, 167-174.
- 12.Srivastava, N. et al. (2009) J Neurophysiol, 102, 1172-1179.
- 13.Iino, Y., & Yoshida, K. (2009) J Neurosci, 29, 5370-5380.
- 14.Wystrach, A. et al. (2016) eLife, 5, e15504.
- 15.Murray, T. et al. (2020) J Exp Biol, 223, jeb210021.
- 16.Clement, L. et al. (2023) Curr Biol, 33, 411-422.
- 17.Wystrach, A. et al. (2019) Anim Cogn, 22, 213-222.
- 18.Baddeley, B. et al. (2012) PLoS Comput Biol, 8, e1002336.
- 19.Wystrach, A. et al. (2011) Front Zool, 8, 21.
- 20.Freas, C. A., & Cheng, K. (2022) Annu Rev Psychol, 73, 217-241.
- 21.Wystrach, A. (2023) bioRxiv, 2023.03.09.531867.
- 22.Lohmann, K. J., & Lohmann, C. M. F. (2019) J Exp Biol, 222(Suppl_1): jeb184077.
- 23.Putman, N. F. et al. (2011) Curr Biol, 21, 463-466.
- 24.Avens, L. et al. (2003) J Exp Mar Biol Ecol, 288, 111-124.
- 25.Buzsáki, G. (2019) The brain from inside out. Oxford University Press.
- 26.VanRullen, R. (2016) Trends Cogn Sci, 20, 723-735.
- 27.Reid, C. R. (in press) Anim Cogn.
- 28.Miller, G. A. et al. (1960) Plans and the structure of behavior. Holt.
- 29.Powers, W. T. (1973) Behavior: The control of perception. Aldine.
- 30.Carver, C. S., & Scheier, M. F. (1998) On the self-regulation of behavior. Cambridge University Press.
- 31.Stanfield, C. L. (2016) Principles of human physiology, Global edition. Pearson.
- *COI:本記事に関連して開示すべき利益相反はない。